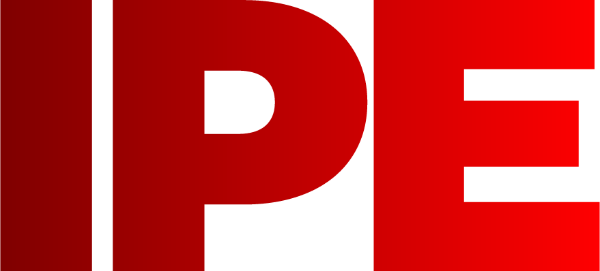
![]() |
Edward Lowton
Editor |
![]() ![]() |
Customisation key to cure
08 January 2020
With personalised medicine and custom prosthetics gaining in popularity Charlotte Stonestreet takes a look at some of the latest technologies to be used in the medical and pharmaceutical sectors
These days, as I write on the cusp of a General Election, the medical and pharmaceutical sectors seem to only be hitting the headlines over the question of whether the NHS will be sacrificed to American pharma giants as part of a post-Brexit trade deal with the US. Whatever your views on the final delivery of healthcare and treatments, and associated social and business models or otherwise thereof, the medical and pharmaceutical sectors are home to some of the most innovative, forward thinking uses of and developments in technology in the world today.
Of course, for almost any operation to remain competitive, automation must be a major consideration. A recent study by the Association for Packaging and Processing technologies (PMMI) has predicted that robots will handle 34 per cent of primary pharmaceutical packaging operations in North America by 2018. An increase in the use of robots is particularly significant in dispensing, sorting, kit assembly and light machine-tending. The advantages include greater speed and accuracy, more flexibility and more reliability.
As the use of the robotics in the pharmaceutical industry increases rapidly, it's important to consider the benefits of the latest technologies for manufacturers, researchers and scientists, asserts Jonathan Wilkins, from obsolete industrial automation parts supplier, EU Automation.
The pharmaceutical industry produces millions of tablets each week, all of which must be carefully scrutinised before being packed and shipped to distributors. During this inspection, drug manufacturers must ensure that the correct amount of medication, with the exact chemical composition and weight are precisely packed into the appropriate containers.
Most pharmaceutical packaging systems use automation to manage bottle orientation, capping, labelling and collation systems. Automation of packaging also requires a system that monitors the operation on a supervisory level, checking for low hopper levels, fallen bottles and low-level supplies.
Until recently, Raman spectroscopy was not widely applied in the pharmaceutical industry. However, in the last few years, developments within the industry coupled with improvements in Raman instrumentation have generated increasing interest in the technology. Raman spectroscopy measures the molecular vibration and rotational energy changes of each tablet, ensuring their chemical compounds are correct before distribution.
This is just one stage of pharmaceutical inspection — automation and machine learning is also applied to ensure that products are packed in the correct bottles and boxes with the correct labelling affixed.
More recently, the pharmaceutical industry has seen the advent of personalised medicine, bringing the industry closer to more precise, predictable healthcare that is customised to the individual patient. Powered by our increased understanding of genetics and genomics, more doctors are providing better disease prevention, more accurate diagnoses, safer drug prescriptions and more effective treatments.
Personalised medicines need automation to reach their full potential. Automation and around-the-clock processing have already contributed towards an environment where personalised medicine is becoming commercially viable. Broader use of genome sequencing for individuals and continued development of drugs proven effective for particular genetic dispositions will continue to accelerate this trend.
Without automation and robotics, scientists often become hampered by the manual testing of individuals' genomes. Today's automated, high-throughput screening is allowing scientists to access a huge amount of data with little manual interaction. This keeps costs low and increases the speed at which the system can analyse gene sequences.
3D printed medicines
Another technology being harnessed in the move towards more personalised medication is 3D printing. In fact, according to Martin Gadsby, Director at Optimal Industrial Technologies, 3D printing is a gamechanger in drug manufacturing, as it gives industries the ability to fully customise medicines - mostly oral solid dosage forms - with realistic production costs. In this way, patients can be treated with more specific dosages and drug combinations that fully address their needs. Even more, this technology empowers pharmaceutical industries to develop medicines with sophisticated bio-functional constructs, which are not achievable with traditional manufacturing practices.
The methods used to produce 3D-printed drugs, or “printlets”, conform to conventional additive manufacturing technologies, such as inkjet printing or fused deposition modelling (FDM). In these systems, the product is often built by depositing highly accurate doses of material layer by layer, until a 3D shape is formed. As a result, reproducibility is a key feature and conventional pharmaceutical manufacturing operations, such as milling, granulating or compressing, do not take place in additive manufacturing.
Instead, in FDM, manufacturers first design a 3D theoretical drug model. Secondly, they choose appropriate printing process parameters, such as layer thickness, extruder diameter, base plate, extruder temperature, printing and extrusion speed. Subsequently, the 3D printing machine reads the models and executes the commands in order to produce the final product.
Therefore, to deliver personalised medicaments with specific drug properties, manufacturers must have a thorough understanding of the different 3D printing processes involved and how they affect the final drug attributes. In this way, they can plan, control and fine-tune the relevant mechanisms to suit.
In order to efficiently monitor the 3D printing line and delivery accurate personalised medicines, it is important to implement a system that can gather, analyse and store analytical and process measurements. Then use these to create and validate models, generate predictions based on these models and provide feedback to the 3D printing system. In this way, manufacturers are empowered with clear actionable insights.
What is called for is a PAT knowledge management tool, a leading solution being Optimal’s synTQ, asserts Gadsby. This is used by over half of the top ten global pharmaceutical manufacturing companies, as it offers a regulatory-compliant, user-friendly platform to detect when a process is moving out of its optimum operating window and correct the relevant CPPs live and in-process.
Customised prosthetics
Another area being revolutionised by the emergence of 3D printing is that of prosthetic devices. With previous technologies prosthetics tended to be heavy, uncomfortable to wear and costly to manufacture. Addressing this, Polish startup Glaze Prosthetics, specialises in customised 3D printed lightweight prosthetic devices that reflect the style, vision, and personality of the wearer.
Because Glaze Prosthetics produces medical-grade equipment, the company needs to ensure that the materials and the production process are fully biocompatible, safe for the user, and free of sharp edges, harsh surfaces, and imperfections. Because each patient has different anatomy and different needs based on their anatomy, Glaze wanted to find a technology that would let them create each product based on individual requirements. Initially, the company began experimenting with 3D printing technologies such as SLS but found that the printing time was slow and costs were high.
Changing to a fishnet-like structure to create the interior of the device rather than printing a solid shell created a device that weighed 70% less than previous devices. Issues with the finish of the product were solved after producing a few iterations with HP Multi Jet Fusion technology, which resulted in Glaze being able to produce parts that were less expensive, lighter, and of a higher quality than previous prosthetics.
With the HP Jet Fusion 4200 3D Printer, Glaze benefited from faster printing times, more durable parts thanks to stronger materials, and lower material costs compared with other 3D printing technologies they had tested.
“It has allowed us to change the scale of production, cut the time of production, and increase the speed of the delivery to the patient,” said Adam Komarowski, Research and Development Leader at Glaze Prosthetics.
Correct Storage
While the manufacture of medical devices such as prothestics has developed, so too have the materials used, often necessitating change in the way businesses handle and store their inventory. For example, Chemstore was recently tasked with improving the storage of Titanium Alloy Powder at the Zimmer Biomet medical manufacturing facility in Bridgend, Wales. Titanium Alloy Powder is flammable and potentially spontaneously combustible, so is imperative that it is stored correctly.
As a solution, Chemstore installed its Firevault. Split into two sections, the Firevault unit has a loading and storage area where pallets of Titanium alloy powder can be accessed by the front by a forklift, and control room accessed via a door on the side, which houses temperature and humidity control, and the fire suppression system.
Fire suppression components are controlled via a panel, which manages the fire detection and manually operated devices. It enables the user to set how the system behaves so that it either automatically extinguishes a fire or allows the user to manage the occurrence. The fire suppression system will only discharge the fire suppression gas if two zones are activated, at a preset time of 60 seconds from the second zone activation. The user can also use the control panel to manually release the gas.
There are two air sampling smoke detectors connected to the fire suppression system, one connected to each zone. Four types of alarm are reported - alert, action, fire one, and fire two.
When the system is about to release the gas a ‘hold off suppressant button’ becomes operational. If activated, the 60 second countdown to gas release will be reset; this can be done as many times as required, however it does not reset the fire discharge sequence but only functions as a delay device. It is mounted both internally and externally.
The right tool
Surgeons performing small bone orthopaedic surgery need total control over the tools they’re using. In an environment where success and failure can be measured by millimetres, there is no margin for error. Portescap has released three new surgical motors designed for orthopaedic saws, drills and reamers. The compact motors offer controllable, precise torque and have been tested to withstand 1,000+ sterilisation cycles.
Orthopaedic surgery is a broad category that treats a range of musculoskeletal conditions arising from trauma, sport injury, bone diseases, degenerative diseases, infections etc. Where procedures are performed on small bones - such as vertebrates or those found in the radiocarpal or talocrural joints – precision is key with every action. The surgical tools used need to deliver power in a smooth and controllable manner to ensure a positive outcome for the patient.
Portescap is at the forefront of sterilisable motor technology; having committed decades to research and development in the medical fields, its motors have been used in millions of surgeries around the world. The latest additions to its range have been designed specifically with small bone orthopaedic applications in mind.
The B0912N1016 Small Bone Motor (9.6 V - 38K rpm), B0912N4023 Small Bone Gearmotor (9.6 V - 1.1K rpm), and B0912N4024 Small Bone Gearmotor (9.6 V - 12.8K rpm) are sterilisable brushless DC (BLDC) motors that provide optimal torque and speed for drills, saws and reamers. They are well-suited for traditional surgical tools - in addition to robotically assisted surgical devices - and can be paired with a Portescap sterilisable controller for battery powered applications.
The motors deliver high peak torque capable of powering through the densest bones and feature customisable voltage to accommodate precise control for the surgeon. The 22 mm diameter motors are lightweight and produce low noise and vibration to improve the ergonomic comfort and control in the hand – essential in long surgeries where fatigue may set in. To ensure reliability and cost efficiency the motors have all been tested to withstand 1,000+ sterilisation cycles as well as exposure to saline and other foreign materials.
- Deep learning brings simplicity to complex AI inspection
- London and the South East an emerging industrial powerhouse
- NPL opens state-of-the-art mechanical test facility
- BEP Solutions to accelerate metal surface R&D
- Mac Plant Construction boosts efficiency and savings with AssetGo
- Distrelec debuts robotics and automation guide
- Midlands manufacturers complain of being locked out of NHS PPE contracts
- Applied intelligence key to efficient order fulfilment
- The PPErfect equipment for staying out of harm’s way
- Kickstart for Manchester SMEs
- No related articles listed